WRITTEN BY: DR KURTIS IRWIN – VP OF GLOBAL CATALYSIS.
INTRODUCTION:
Air pollution is a pressing concern with significant implications for human health and the environment. As engineers strive to address climate change and reduce carbon emissions, the role of aftertreatment systems to clean and decarbonise the air has become increasingly crucial. This blog examines the technical significance of aftertreatment systems, focusing on their engineering impact in improving air quality and reducing emissions.
Understanding Aftertreatment Systems:
Aftertreatment systems are advanced engineering technologies integrated into vehicles, industrial processes, and power plants to mitigate harmful emissions. These systems involve a range of components and technologies designed to treat exhaust gases before they are released into the atmosphere.
Aftertreatment systems encompass diverse technologies, and in the context of gasoline engines, the Three-Way Catalyst (TWC) holds a pivotal role. TWCs are sophisticated devices designed to simultaneously reduce three major pollutants—nitrogen oxides (NOx), carbon monoxide (CO), and unburned hydrocarbons (HC)—through catalytic reactions.
In modern engine systems, TWCs are often part of a comprehensive aftertreatment system that may include additional components such as gasoline particulate filters (GPFs) and advanced sensors. Integrating these technologies ensures a holistic approach to emissions reduction and compliance with stringent environmental regulations.
Understanding the intricacies of Three-Way Catalysts is crucial for engineers seeking to optimize their performance within aftertreatment systems. The delicate balance required for their operation and their integration into the broader context of emissions control highlight the complex engineering considerations in achieving cleaner air through gasoline engine technologies.
Particulate matter emissions pose a significant challenge in air pollution management. For gasoline engines, aftertreatment systems employ gasoline particulate filters (GPFs) to capture and remove particulate matter from the exhaust stream. GPFs leverage porous materials and advanced filtration mechanisms to efficiently trap and reduce particulate emissions, significantly improving air quality.
Reducing nitrogen oxide emissions is crucial for curbing air pollution. Aftertreatment systems for gasoline engines employ various engineering techniques such as lean NOx traps (LNTs) and selective catalytic reduction (SCR) systems. LNTs use catalysts to store nitrogen oxides during lean engine operation and periodically release and convert them to harmless gases under rich conditions. SCR systems utilise catalysts to facilitate the selective reduction of nitrogen oxides into nitrogen and water vapor, minimising their environmental impact.
Efforts to address greenhouse gas emissions are paramount in combating climate change. Aftertreatment systems for gasoline engines contribute indirectly to decarbonising the air by enhancing engine efficiency and reducing fuel consumption. Advanced engineering technologies like direct injection, turbocharging, and hybrid powertrains, combined with aftertreatment systems, optimise combustion processes and reduce carbon dioxide emissions, aiding in the fight against global warming.
Engineering implications on performance and efficiency:
Aftertreatment systems must strike a balance between emissions reduction and maintaining optimal engine performance. Engineers face the challenge of designing aftertreatment systems that minimise backpressure and thermal losses while achieving efficient emissions treatment. Innovative catalyst formulations, improved materials, and advanced control strategies enable engineers to optimise aftertreatment systems, ensuring both emissions compliance and optimal engine performance.
Technological advancements and future trends:
The field of aftertreatment systems for gasoline engines is continuously evolving through technological advancements and research efforts. Engineers are exploring novel catalyst materials, advanced sensors, and system integration strategies to enhance efficiency, durability, and cost-effectiveness. Additionally, the integration of electrification technologies and hybrid powertrains presents opportunities for further optimising aftertreatment systems and achieving even cleaner and more sustainable emissions performance.
“In the context of aftertreatment systems, catalyst durability is a critical aspect that engineers must consider. Catalysts play a vital role in facilitating the conversion of harmful emissions into less harmful substances.”
However, over time, catalysts can degrade due to various factors such as exposure to high temperatures, chemical reactions, and contaminants present in the exhaust gases.
Understanding catalyst degradation is of paramount importance for maintaining the long-term effectiveness of aftertreatment systems. Engineers strive to analyse and characterise catalyst aging mechanisms to design more durable catalyst formulations. By understanding the catalyst degradation processes, engineers can develop strategies to mitigate degradation and extend the lifespan of catalysts, thereby ensuring sustained performance and emissions reduction efficiency.
Factors such as thermal stability, poisoning by sulphur compounds or metals, and the impact of catalyst washcoat ageing need to be thoroughly studied and accounted for during the design and optimisation of aftertreatment systems. This involves conducting accelerated ageing tests, real-world driving simulations, and utilising advanced characterisation techniques to monitor catalyst performance over time.
Moreover, advanced diagnostics and monitoring systems are being developed to provide real-time feedback on catalyst health and efficiency. These systems enable early detection of catalyst degradation, allowing for timely maintenance or replacement, thus minimising the risk of emissions non-compliance and ensuring optimal system performance.
By addressing catalyst durability and understanding degradation processes, engineers can enhance the reliability and longevity of aftertreatment systems. This proactive approach not only optimises emissions control but also helps reduce the overall cost of ownership by maximising the lifespan of expensive catalytic components.
In conclusion:
Catalyst durability is a crucial consideration in aftertreatment systems. Understanding catalyst degradation mechanisms empowers engineers to design more robust systems and develop strategies to mitigate degradation effects. By employing advanced diagnostics and monitoring systems, engineers can detect and address catalyst ageing in a timely manner, ensuring sustained performance and emissions reduction efficiency. Ultimately, a comprehensive understanding of catalyst durability contributes to the long-term success and effectiveness of aftertreatment systems, promoting cleaner air and a healthier environment.
From an engineering perspective, aftertreatment systems are critical to clean and decarbonise the air, not only for diesel engines but also for gasoline engines. Through effective control of particulate matter, nitrogen oxides, and carbon dioxide emissions, these systems significantly contribute to improving air quality and reducing the environmental impact of gasoline engines. Engineers continue to drive advancements in aftertreatment technologies, ensuring emissions compliance, optimal engine performance, and a sustainable future for cleaner air.
CATAGEN’s purpose is to clean and decarbonise the air.
Bio – Dr Kurtis Irwin
Kurtis is the VP of Global Catalysis at CATAGEN. He has over 10 years experience specialising in after-treatment systems and catalysis.
He is a Doctor of Philosophy (Ph.D.) focusing on mechanical engineering and he was recently awarded the UKRI Future Leader Fellowship award.
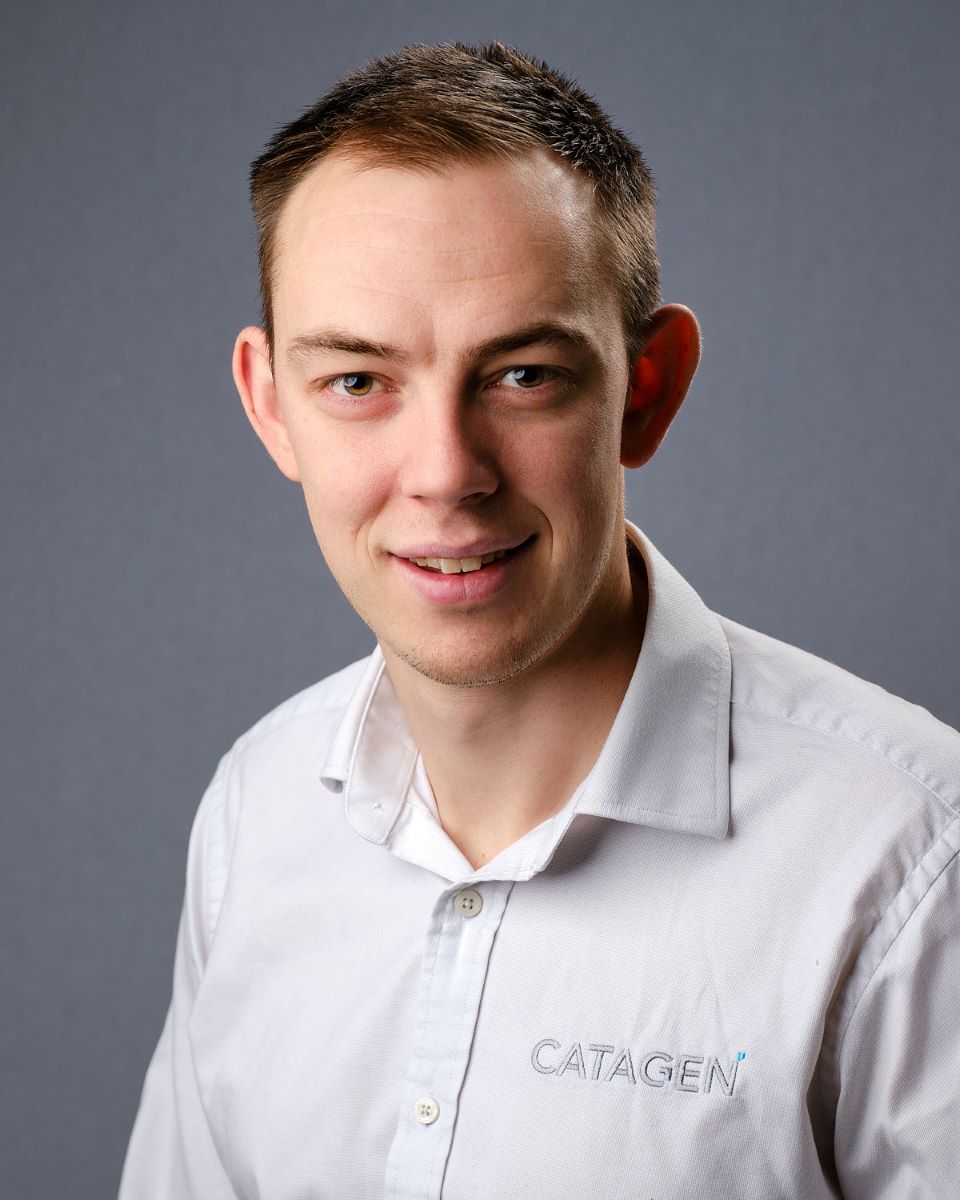
WHY OEM’s PARTNER WITH CATAGEN
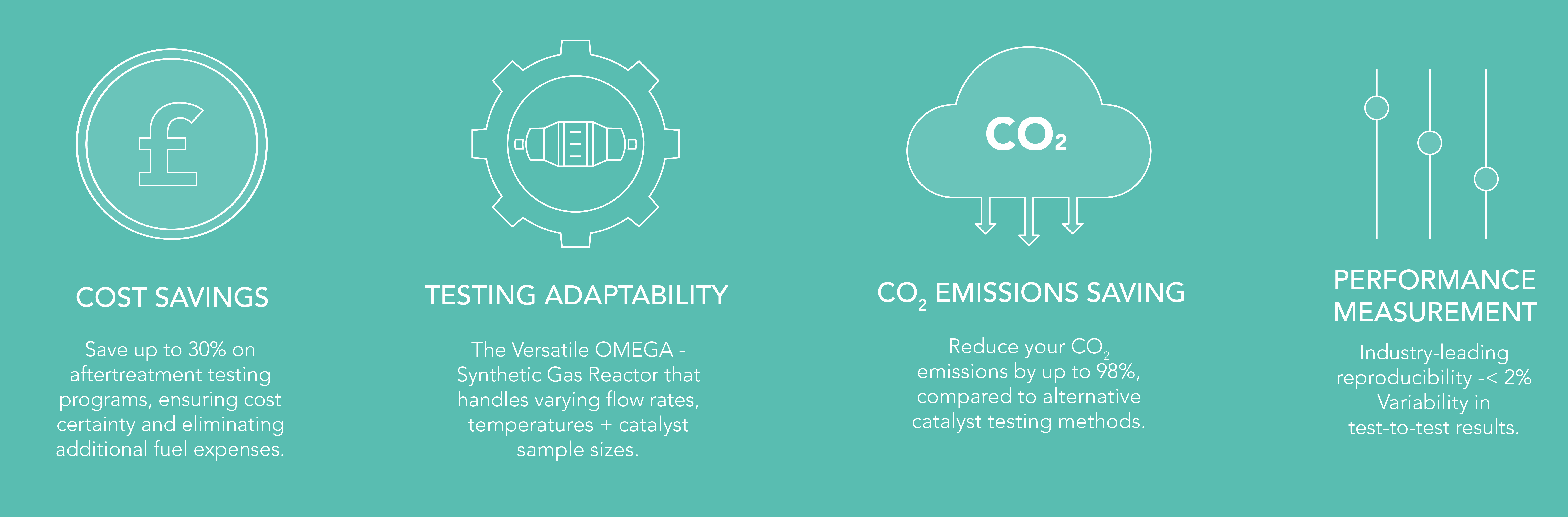